Current Research
Atmospheric River
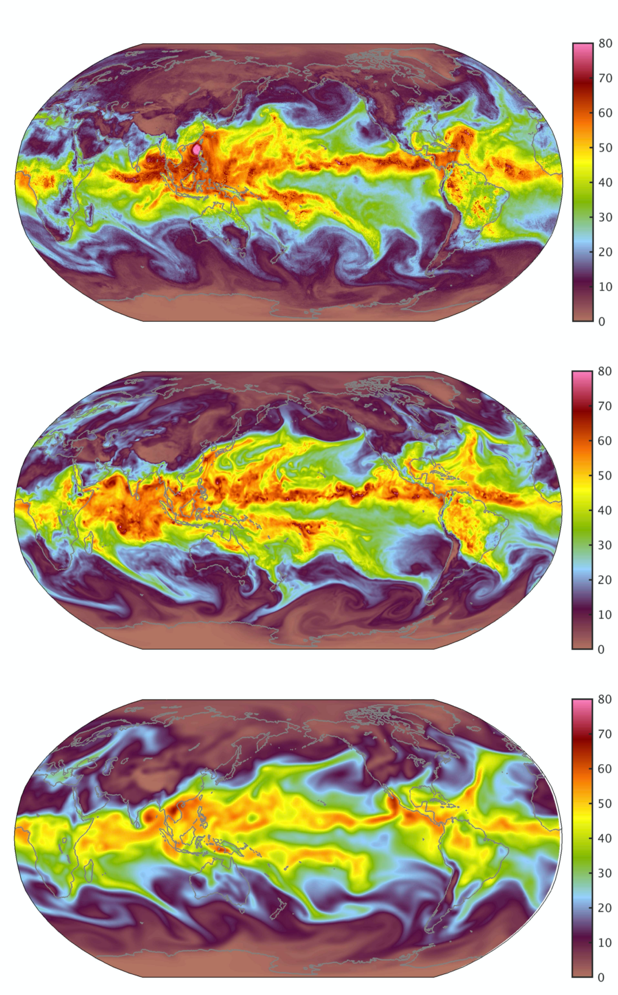
Overview
Atmospheric rivers (ARs) are plumes of intense water vapor transport emanating from the atmospheric moisture pool, responsible for over 90% of poleward water vapor transport in the midlatitudes.
Why this research is relevant?
ARs have been recognized as one of the most important sources of extreme hydroclimate events in the global extratropics, capable of producing torrential rains and floods when making landfall over regions of elevated orography, such as the West Coast of North America. Some of the most severe river floods in California were associated with ARs. A recent global analysis of ARs’ role in driving hydrological extremes found that ARs can contribute not only to extreme floods in many major drainage basins, but also to drought occurrence when ARs are inactive. Therefore, developing and improving the capability of predicting ARs at subseasonal-to-seasonal (S2S) time scales, especially landfalling ARs, can have important implications for water resource management, flood control, and drought relief.
What are the challenges of the research?
Weather and climate models still have difficulty simulating and predicting landfalling ARs and associated extreme precipitation, highlighting the need to better understand AR dynamics. Although increasing model resolution in current weather forecast models can lead to forecast bias reduction of overall AR occurrence and intensity, the timing and location of landfalling ARs, as well as their precipitation impact, are notoriously difficult to predict, underscoring the importance of a better understanding of ARs’ dynamics and their predictability sources. There is a concerted, ongoing research effort to understand and quantify predictability and uncertainty in forecasting AR, including the community-driven Atmospheric River Tracking Method Intercomparison Project (ARTMIP) – dedicated to evaluate impacts of AR detection algorithms on various science questions.What we are working on now?
At iHESP, we are using high-resolution climate models and observations to investigate ARs’ dynamics and their predictability sources. Our modeling results show that AR simulations are significantly improved by increasing model resolution (see figure). One ongoing research activity focuses on the role of mesoscale sea-surface temperature (SST) anomalies along western boundary current regimes, such as the Kuroshio and Gulf Stream, in influencing landfalling ARs and related heavy precipitation. We are also working to address the issue of how ARs will respond to global warming. Additionally, we have developed a new AR tracking algorithm to reduce uncertainties in identifying and detecting ARs.
Coupled Data Assimilation
Achieving the goal of generating skillful forecasts for climate variations would be of great importance to our society. The coupling between various earth system components (the ocean, atmosphere, and land) plays a critical role on climate variability. Therefore the development of coupled data assimilation (CDA) for coupled general circulation models is emerging as an important strategy for improving the forecasts. A state-of-the-art CDA scheme should combine observations from different model components with a couple general circulation models to provide accurate model initial conditions for the climate forecast.
Currently we have implemented an online ensemble Kalman filter (EnKF) based data assimilation capability in the ocean components of R-CESM. We are testing the capacity using pseudo-observations and real observations with the the domain configuration of the Gulf of Mexico at eddy-resolving resolution. In the next phase of this research, we will implement the online data assimilation capability into the atmospheric component of R-CESM and configure a CDA system for the Gulf of Mexico.
Decadal Prediction
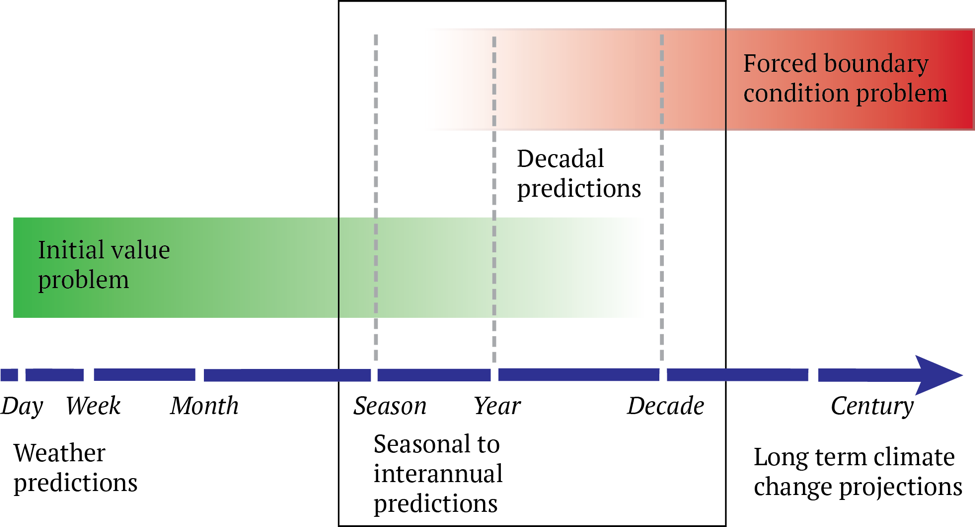
While seasonal climate prediction has become operational since the 1990s, studies in the past decade have shown that observation-based initialization of coupled general circulation model (CGCM) simulations of the last half-century can significantly enhance predictive capacity on time scales from a year to a decade or more in advance. First implemented in Phase 5 of the Coupled Model Intercomparison Project (CMIP5) in the early 2010s, an extensive set of coordinated decadal prediction experiments is now included in the latest CMIP6. These experiments provide a framework for testing the benefits of historical initialization, in addition to prescribed external forcings, for decadal climate outlooks that are sensitive to both initial conditions and forced boundary conditions (see figure). This framework involves running fully-coupled hindcast (i.e., retrospective forecast) ensembles initialized using observationally based state information at prescribed intervals over the historical period; these hindcasts are then verified against observations and compared to uninitialized, free-running simulations to determine both overall skill and the benefits of initialization.
Using the standard-resolution Community Earth System Model (CESM), a large ensemble of decadal predictions (CESM-DPLE) was carried out. CESM-DPLE is composed of 40 member ensembles, with each ensemble initialized on November 1 between the years 1954 and 2017 (for a total of 64 start dates), and integrated for 122 months. This prediction ensemble exhibits quite promising levels of skill for many different fields, across a broad range of forecast lead times up to decadal scales, both in the ocean and over land.
At iHESP, we are investigating the benefits and challenges of decadal climate prediction at high-resolution. We are conducting a subset of CESM-DPLE experiments, which consists of an ensemble of 10 (rather than 40) high resolution CESM (CESM-HR) prediction runs for a 40-year period from 1979 to 2018. Each prediction run will be integrated for 5 years (rather than 10 years), starting from November 1 of every other year (rather than every year) during the 40-year period. Although the ensemble size of the CESM-HR prediction runs is smaller than that of the low-resolution CESM-DPLE, we believe that this ensemble size is sufficiently large to analyze differences between the high- and low-resolution CESM predictions and determine the benefits of high-resolution climate predictions.
Hurricane Simulation
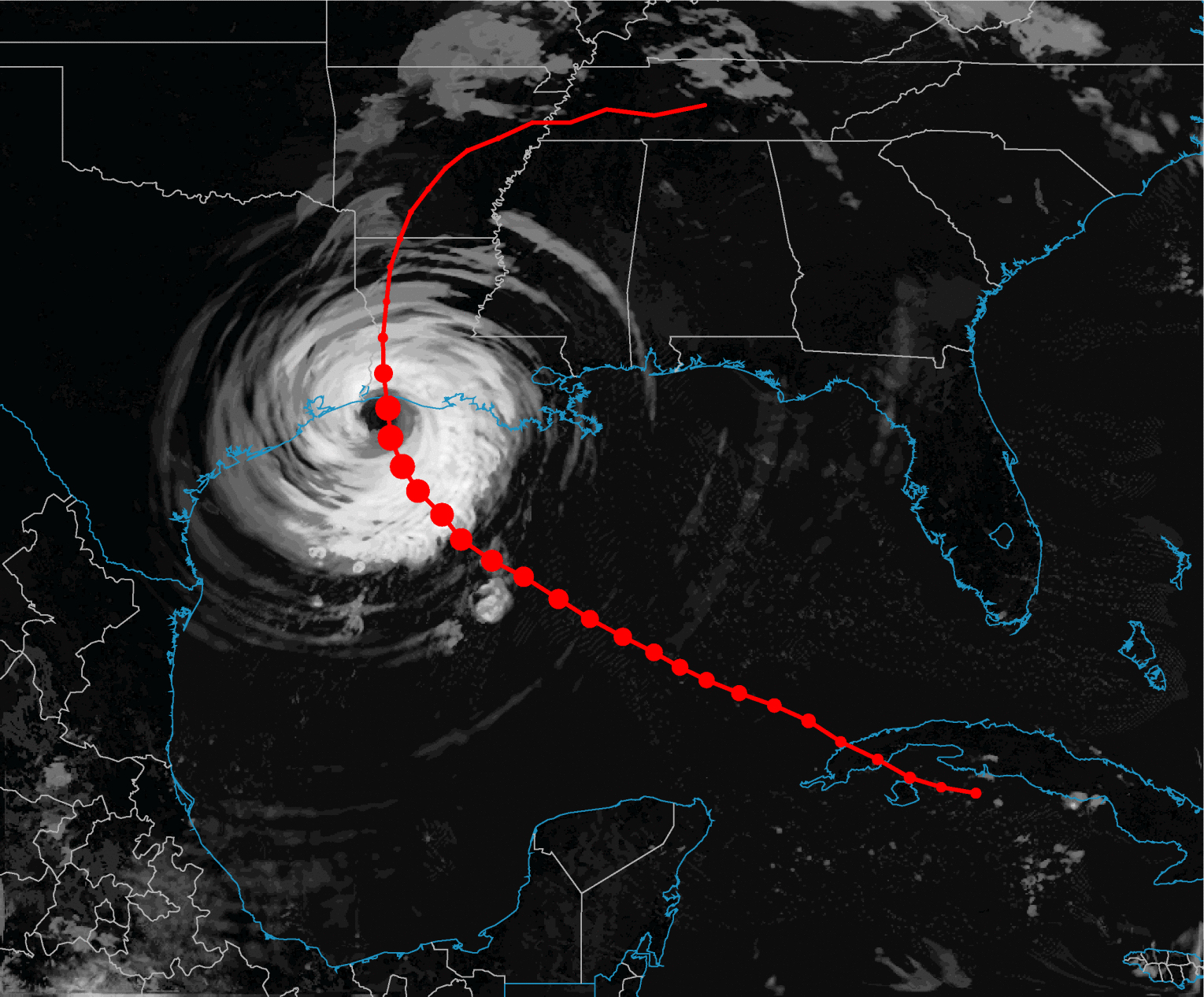
Hurricanes cause multitude of threats to the people and property and hence it is of utmost importance to understand their dynamics and improve the accuracy of their forecasts. While the accuracy of hurricane track forecast has been improved significantly in the recent decades, intensity forecast proven to be challenging. The physical processes behind the formation and intensification of hurricanes are not well understood yet. High-resolution regional coupled models like R-CESM offers a unique opportunity to study the ocean-atmosphere interaction during different stages of hurricanes. Using a range of model simulations, researchers at iHESP are trying to learn about various aspects of hurricanes in order to: i) assess the impact of model physics and parameters on the hurricane simulation ii) improve the accuracy of hurricane simulations in the R-CESM and iii) assess the impact of ocean heat content and mesoscale eddies on the rapid intensification of the hurricanes. The ongoing coupled data assimilation research at iHESP using the R-CESM framework has the potential to improve the dynamical model based hurricane forecasting in the future.